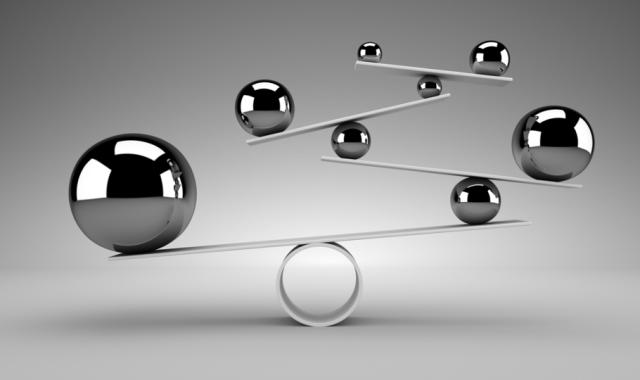
The current state of oil and gas E&P finds operators continuing to move into more challenging drilling and production environments, where recoverable volumes might be low compared to the complexity of the reservoir and the high costs of production. Geomechanical challenges in the form of wellbore and fault instability, surface subsidence, and sand production might pose high risks to an operator’s field development plans and threaten the profitability of each well if not properly assessed and resolved.
Geomechanical modeling is not a “one size fits all” proposition.
Advances in geomechanical modeling have helped operators develop better well plans and define safer operating windows to optimize drilling performance, minimize drilling risks and improve safety. However, geomechanical modeling is not a “one size fits all” proposition, and operators do not need to select the most technically complex, laborious and costly model if the situation does not require it. Implementing the right geomechanical model, one with a scale and sophistication that matches the complexity of the reservoir under study, is critical to identifying rock mechanics challenges while also arriving at an optimized well plan without wasting time, computational power and software license costs.
This article compares different geomechanical models built using the Baker Hughes JewelSuite Geomechanics software application. The models are analyzed for their accuracy and resource efficiency in different structural settings.
Model Fundamentals
The JewelSuite Geomechanics application builds precise models ranging in scope and complexity from 1-D well-centric to 4-D full-field geomechanical models, giving the operator greater insight to optimize well plans. The application’s 1-D model module uses log data and core sample data to build preproduction workflows that include lithology, overburden, pore pressures, stress orientation and magnitude, mapping, wellbore stability, and fracture gradients. Stress orientation is identified by interpreting caliper and image logs, while stress magnitudes and pore pressures are found from rock modeling and well tests such as leakoff or minifrack tests. The software lets the user display drilling events, manage multiple wells, depth-stretch logs and formation tops, manipulate logs graphically, analyze local correlations and generate customizable reports.
For more sophisticated interpretations, the application’s 3-D model module builds accurate 3-D static reservoir-scale geomechanical models from 1-D models and a 3-D structural model. For this methodology a 3-D grid is populated with the properties of the 1-D geomechanical model using geostatistical methods. Vertical stresses, pore pressures and horizontal stresses are then calculated by adopting a similar workflow to those used in 1-D models. In this case, calculations are not performed along a well but along the vertical orthogonal pillars of the grid.
While a 3-D geomechanical model is effective at capturing lateral variations in formation properties, it is less applicable in more geologically complex situations. Such settings include a salt dome where the formation cannot withstand differential stresses, situations when reservoir pressure changes over time or in reservoirs experiencing varying depletion in compartments.
These complex geological settings are resolved through numerical techniques such as finite element modeling. Unlike with 3-D models, these techniques simulate rather than calculate the stresses on a reservoir scale. Finite element modeling enables the simulation of a complex stress field with changing boundary conditions over multiple time steps. The resulting 4-D geomechanical models allow better understanding and mitigation of the effects of geomechanical changes over the life of the field.
Comparing Geomechanical Models
The geomechanics software application was used in a study aimed at comparing three different geomechanical models— 1-D, 3-D and 4-D—for their accuracy in estimating the state of stress in a real-world simulation. A synthetic bathymetrical map was created to represent a general coastal setting of a continental shelf containing a 1,400-m (4,600- ft) deep submarine canyon. The full seafloor area covered 180 km by 150 km (112 miles by 93 miles).
To shorten the computation time required for the 3-D and 4-D reservoir-scale models, two smaller areas with lateral dimensions of about 32 km by 32 km (20 miles by 20 miles) were selected for analysis. One of the areas covers part of the continental shelf and the other part of the submarine canyon.
Using the software application, researchers created multiple 1-D geomechanical models using well data; these 1-D models were subsequently used to construct 3-D geomechanical models for both the submarine canyon and the continental shelf area. Two 4-D geomechanical models also were created using the structural framework of the 3-D model, rock properties and knowledge on the initial stress state of the 1-D geomechanical models. Then the stress state along the well paths was extracted from the 3-D and 4-D geomechanical models and compared.
Results and Discussion
A comparison of the pressures and stresses for four different wells—two vertical wells and two deviated wells— generated by the 1-D and 3-D geomechanical models is presented in Figure 1. Two of the wells (denoted as Wells A and B) were located in the submarine canyon area, while the other two (Wells E and F) were on the continental shelf.
FIGURE 1. This table shows a comparison of pressures (Pp) and stresses (Shmin, SHmax, Sv) in the 1-D and 3-D geomechanical models along trajectories of Wells A, B, E and F. The 1-D and 3-D model results correlate strongly for Wells A, E and F, with the 3-D results (darker color and blocky) essentially overlaid on the 1-D results (light colored). (Source: Baker Hughes)
The results indicate a good match for Wells A, E and F using the 1-D and 3-D models but a significant difference for Well B, which was an inclined well with a long lateral section. This difference is likely due to the fact that Well B’s lateral was located below the submarine canyon, which meant that there was less rock and therefore less overburden weighing down on it. As a result, much less overburden was used for the vertical integration in the 3-D grid, which led to a lower vertical stress.
Figure 2 compares the stress difference in cross sections between the 3-D and 4-D models. The models match closely for the continental shelf but show a greater disparity for the submarine canyon. This is because the 3-D model calculates the stresses along the vertical stacks of grid cells without considering lateral effects. The 4-D model simulates the stress state while accounting for the stress effect of the canyon walls.
FIGURE 2. Ratios are shown between the principal effective stress magnitudes in the 3-D and 4-D geomechanical models for the continental shelf (left) and submarine canyon (right). From top to bottom are the ratios of the maximum principal effective stress, σ1 (about vertical), the intermediate, σ2 (about horizontal) and the minimum, σ3 (about horizontal). (Source: Baker Hughes)
This study supports the claim that accurate answers do not always come from the most complex modeling solution. While a 4-D model might be required to accurately simulate the state of stress in a complex submarine canyon setting, a 1-D wellbore-scale geomechanical model can be an efficient way to model stress states in geological settings that do not experience dynamic processes like depletion or salt creep. And by not automatically resorting to building 3-D or 4-D reservoir- scale models for every well, an operator can still gain the subsurface insight required to optimize well plans but in less time and at lower costs. Additionally, it can save time for better analysis of the results, adding uncertainty assessment to the model and/or doing a sensitivity analysis.
Recommended Reading
E&P Highlights: July 22, 2024
2024-07-22 - Here's a roundup of the latest E&P headlines, with LLOG acquiring 41 blocks in the Gulf of Mexico and Saipem securing $500 million in contracts from Saudi Aramco.
Seadrill to Adopt Oil States’ Offshore MPD Technology
2024-09-17 - As part of their collaboration, Seadrill will be adopting Oil States International’s managed pressure drilling integrated riser joints in its offshore drilling operations.
E&P Highlights: Sept. 16, 2024
2024-09-16 - Here’s a roundup of the latest E&P headlines, with an update on Hurricane Francine and a major contract between Saipem and QatarEnergy.
E&P Highlights: Aug. 26, 2024
2024-08-26 - Here’s a roundup of the latest E&P headlines, with Ovintiv considering selling its Uinta assets and drilling operations beginning at the Anchois project offshore Morocco.
E&P Highlights: Sep. 2, 2024
2024-09-03 - Here's a roundup of the latest E&P headlines, with Valeura increasing production at their Nong Yao C development and Oceaneering securing several contracts in the U.K. North Sea.
Comments
Add new comment
This conversation is moderated according to Hart Energy community rules. Please read the rules before joining the discussion. If you’re experiencing any technical problems, please contact our customer care team.